Three ways to break a sugar chain
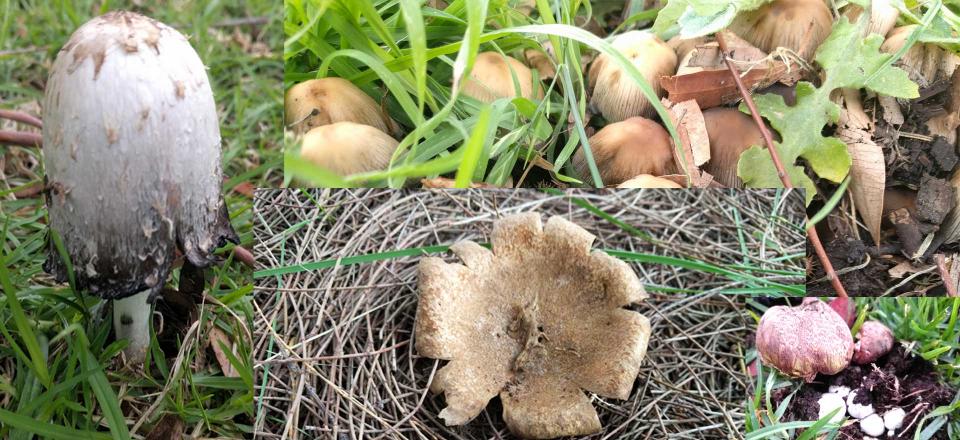
Three ways to break a sugar chain
By Spencer Williams and Florienne Loder
As autumn rains moisten the soil mushrooms sprout amid the leaf litter on our nature strips and in our parks and forests. These fungi contribute to the process of decomposition, which is part the cycle of life: death, decay and decomposition are bookends to new life and growth. Dead organic matter is recycled and provides nutrients for new plant growth.
Under a microscope, you can discover a multitude of microorganisms, bacteria and fungi living within a teaspoon of compost. Our gut also contains beneficial bacteria and fungi that break down the carbohydrates that we consume in our diets.
You would be forgiven for thinking that the products of life are temporary, built from delicate materials that are easily destroyed.
You would be forgiven for thinking that the products of life are temporary, built from delicate materials that are easily destroyed. Yet a tree can live for 4700 years, like the bristlecone pine tree (Pinus longaeva) from California's White Mountain range, nicknamed ‘Methuselah.’ And intact wooden boats were discovered in the pyramid of Giza, dating from c. 2580 – 2503 BC.
The ‘stuff’ of nature is composed of complex carbohydrates. Complex carbohydrates are long chains of sugar molecules made up of smaller – ‘monosaccharide’ units – stringed together like a pearl necklace and linked by a particular kind of chemical bond: Carbon bound to an Oxygen atom, called a ‘Glycosidic Bond’.
Complex carbohydrates are the ubiquitous molecules of life, more abundant that any other biopolymer on earth. And the bonds that hold them together – the glycosidic bonds – are among the strongest bonds of nature.
Discovery of a new strategy for breaking down carbohydrates
Nature has ‘chosen’ wisely, because glycosidic bonds have an estimated half-life of billions of years.
If that is the case, how are these strong bonds so easily broken down?
To break down these carbohydrate chains and liberate monosaccharides, like glucose from complex carbohydrates, Nature has evolved powerful protein catalysts, enzymes, to accelerate chemical reactions that cleave these bonds.
These enzyme catalysts are termed ‘glycosidases’, and they allow the bacteria in our gut to digest complex carbohydrates, and fungi to feed on rotting trees.
How do glycosidases break such stable bonds? Scientists have made intensive efforts dating back nearly 70 years to explain how glycosidases work in molecular detail. The surprising outcome of all this work is that of the hundreds of thousands (and probably millions) of glycosidases, essentially only two chemical mechanisms have been discovered.
Two ways to break a bond...
The most common mechanism is one in which the glycosidase enzyme uses two strategically located ‘amino acid residues’, - the unique chemical signatures on amino acids - to chemically cleave the bond. These molecules act like a pair of clippers that snip and cut the bond. This approach is used in a famous enzyme (lysozyme) found in egg-white that protects the chicken embryo from bacteria by cutting their cell walls.
The second mechanism needs uses one amino acid residue, and instead uses another type of chemical unit, an ‘amide group’ on the glycoside (such as in crab chitin) as the second residue. This is used by glycosidases called chitinases to degrade crab exoskeletons allowing them to molt. These are now textbook mechanisms studied in undergraduate courses.
A third way…
But a new discovery has revealed a third way: forming a three-membered ring.
The orthodoxy of just two mechanisms has now been challenged through the new discovery of a third way that nature breaks glycosidic bonds.
A multinational team of scientists, including Bio21’s Professor Spencer Williams, has discovered a special group of enzymes that instead use another type of chemical structure, a sugar hydroxyl and that forms a three membered ring (an epoxide) intermediate.
"It is wondrous to learn that nature long ago learnt how to sustain life using a reaction pathway that we had thought was nothing more than a curiosity" commented Professor Spencer Williams.
–Professor Gideon Davies added “As new glycosidases are being discovered, we think that this mechanism will turn out to be more widespread than we now think.”
– “By discovering how nature works, we can learn to mimic its strategies to develop new enzymes for industrial applications,” argues Professor Carme Rovira.
The study, published in the journal ACS Central Science (“An epoxide intermediate in glycosidase catalysis”), involves research teams from three different continents and five countries, led by Spencer Williams, University of Melbourne (Australia); Gideon Davies, University of York (UK), Carme Rovira, University of Barcelona (Spain), …, Matthieu Sollogoub (France), Andrew Bennett, Simon Fraser University (Canada), Jesus-Jimenez Barbero and Oscar Millet, CIC Biogune (Spain).
Intriguingly, this new biological mechanism had been known to occur for over a century when carbohydrates were treated with a strong base, like caustic soda (sodium hydroxide). It is remarkable that this old reaction that had until now been observed only under such forcing chemical conditions has been coopted by nature and used by organisms to cleave sugars.
This new mechanism was studied in a bacterial model protein but is also believed to occur within our bodies in a special processing enzyme called ‘endomannosidase’ that is involved in modifying sugars that are often attached to our proteins.
The study involved a battery of advanced techniques: protein crystallography, chemical biology and spectroscopy and computational biology.
Because biomass is so abundant there are numerous industrial applications of glycosidases in the food, paper and textile industries (e.g. to restore brightness in cotton fabrics; clarification of juices; bread-baking; and even fabric brighteners), for the production of carbon-neutral biofuels by breaking down wood waste, and even in the gas & fuel industry to help natural gas flow from deep underground. Other glycosidases, such as the neuraminidases present in the capsid of the influenza virus, are drug targets for the common flu and genetic disorders.
Media Enquiries:
Professor Spencer Williams is available for comment.
Please contact:
Florienne Loder
Communicationsa and Engagement Advisor
0404 230 006