Everyday quantum mechanics and the study of cell membranes
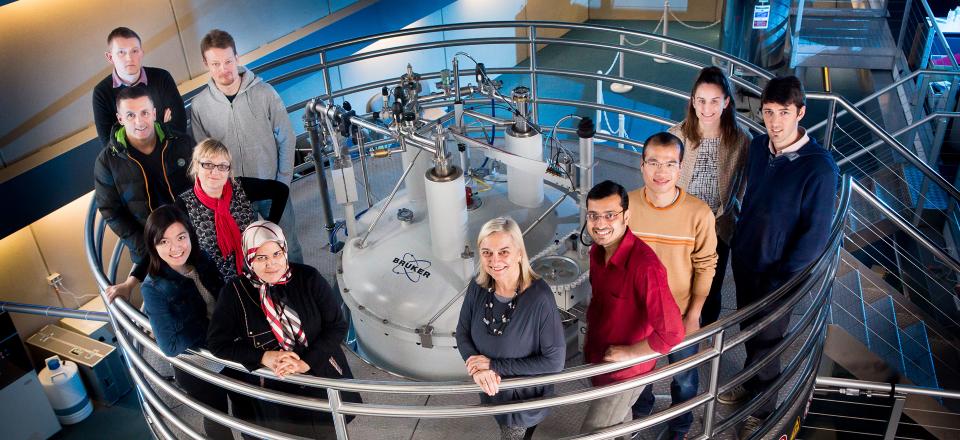
A precious cargo arrived from Bruker at Bio21 Institute in wooden-sealed boxes on the 7 February 2015.
Weighing 560 kgs (798 kg filled plus 380 kg accessories) and needing to be kept upright, it was a precarious challenge to unload the $720,000 solid-state nuclear magnetic resonance (NMR) spectrometer at Bio21’s loading dock.
In the course of a few weeks it was unpacked, assembled and positioned in its new home in the Magnetic Resonance Facility at the Bio21 Institute.
Professor Frances Separovic had lodged the successful application Australian Research Council (LIEF) infrastructure funding scheme to acquire the solid-state NMR spectrometer for Bio21’s collection.
The Bio21 Magnetic Resonance Facility houses nine magnetic resonance machines that are available for use by the scientific community on a user-pays system. It is one of Australia’s leading magnetic resonance centres.
These remarkable machines make it possible to analyse the building blocks of matter. They are used to determine the structure of molecules, ranging from small chemicals to proteins and nucleic acids.
“An NMR spectrometer tells you how far apart the atoms are; their distance and how they are connected with each other; what’s connected to what, through what kind of bond,” explains Professor Separovic.
Nuclear Magnetic Resonance is commonly used by organic synthetic chemists to validate that they have successfully created certain compounds.
Its name yields clues as to how it works. ‘Magnetic’ refers to the fact that these machines use powerful superconducting magnets: niobium-tin coils, that when extended, reach up to 100 kilometres in length. These coils are compressed inside a sealed metal chamber, encased in a vacuum and kept at a temperature of -269°C.
Radio frequency waves excite the nuclei in atoms and molecules, causing them to resonate and give off a unique frequency, which can be used to identify them. The data appear as spectral readings on a graph.
“NMR is everyday quantum mechanics – it’s beautiful,” says Frances Separovic, who has had a lifelong passion for the subject.
Professor Separovic is interested in membrane-bound antimicrobial peptides, small proteins, for example, on frog skin that protect against infection.
Membranes are structures that form in water to separate the inside from the outside. It is relatively easy to create an artificial membrane: simply add detergent-like lipid molecules to water. These surfactant molecules align with their ‘hydrophobic’ water-hating ‘tails’ facing inwards and their ‘hydrophilic’ water-loving ‘heads’ facing outwards. More often than not they will form circular structures called vesicles. Biological membranes such as the cell membrane are structured according to this principle.
There are a number of proteins that span cell membranes and Frances Separovic is interested in these, particularly where they have some antimicrobial function.
Up until now, it’s been very difficult to study these proteins. The tools available to analyse their structures at atomistic resolution - X-ray crystallography and solution-state NMR – have their limitations.
X-ray crystallography makes it possible to determine the structure of molecules in their crystal form. However, it is difficult to crystallize certain molecules, particularly those in biological membranes. Also, when crystallized, the crystal structure may not represent a protein’s naturally occurring physiological state. In X-ray crystallography, samples are typically frozen at -140°C.
Alternatively, molecules can be analysed using liquid-state NMR but when molecules are dissolved in solution, they tumble around in the liquid at high speeds.
“However, a biological membrane is neither completely solid nor liquid: it’s a liquid crystal,” explains Professor Separovic.
“The solid-state NMR spectrometer makes it possible to determine protein structures in a more physiological environment, at room temperature,” she says, “and the capability to determine membrane protein structure and interactions in mammalian and bacterial cells.”
This is revolutionary for the study of membrane-bound proteins and their interactions, which in turn informs drug design that targets these structures. Understanding interactions in cell membranes is central to understanding biochemical mechanisms and disease states and to develop new drug treatments.
In collaboration with other Bio21 researchers, Professor Separovic is also using the solid-state NMR to study the aggregation of amyloid plaques that is a symptom of Alzheimer’s disease.
While the potential outcomes of her research range from the development of new and improved antibiotics to an increased understanding of the causes, and thus potential treatments, of Alzheimer’s disease, Professor Separovic explains what drives her:
“Deep down, I just want to know how things work. Once you know how something works, then you can fix it, or make it better,” Frances explains.
The Bio21 Institute’s new solid-state NMR will allow this investigation at a previously impossible level of detail, supporting researchers like Professor Separovic and her team looking to push their boundaries and explore new frontiers.
Access: The solid state NMR spectrometer is available and can be booked through the Magnetic Resonance booking system.
By Florienne Loder, External Relations Officer, Bio21 Institute.